Introduction
Tractive power and energy consumption are key performance indicators for vehicles, especially in the context of increasing environmental concerns and the push towards sustainable mobility. This article delves into the intricate relationship between tractive power and energy consumption, comparing different vehicle types and discussing strategies to optimize energy efficiency.
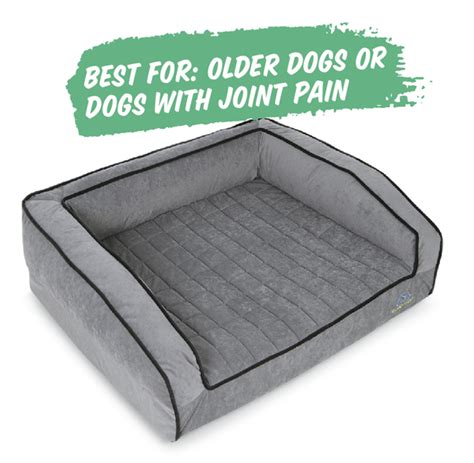
Tractive Power
Tractive power, measured in newtons (N), represents the force required to move a vehicle forward. Factors influencing tractive power include:
- Vehicle weight
- Rolling resistance
- Aerodynamic drag
- Gradient (slope)
Energy Consumption
Energy consumption, typically expressed in kilowatt-hours per 100 kilometers (kWh/100 km), quantifies the amount of energy required to move a vehicle a specific distance. It is influenced by:
- Tractive power
- Vehicle efficiency
- Driving behavior
Tractive Power VS Energy Consumption
The relationship between tractive power and energy consumption is complex and interdependent.
- Higher tractive power demands more energy, as overcoming resistance requires increased power output.
- Reduced energy consumption results from minimizing tractive power through measures such as lightweight design, reduced rolling resistance, and improved aerodynamics.
Electric Vehicles VS Internal Combustion Engine Vehicles
Electric vehicles (EVs) and internal combustion engine (ICE) vehicles exhibit distinct characteristics in terms of tractive power and energy consumption:
Feature | Electric Vehicles | Internal Combustion Engine Vehicles |
---|---|---|
Tractive power | Instantaneous torque provides high tractive power | Power output limited by engine speed and torque |
Energy consumption | Generally lower, especially at low speeds | Higher, due to fuel consumption and heat loss |
Strategies for Optimizing Energy Efficiency
Optimizing energy efficiency involves a multifaceted approach:
- Vehicle design: Lightweight materials, aerodynamic enhancements, and efficient powertrains reduce tractive power.
- Advanced technologies: Regenerative braking and energy-saving modes recover and conserve energy.
- Driving habits: Smooth acceleration, anticipatory braking, and maintaining optimal speed minimize energy consumption.
Case Study: Tesla Model 3
The Tesla Model 3 stands out as a prime example of energy efficiency optimization:
- Low rolling resistance: Special tires minimize friction.
- Aerodynamic design: A sleek shape reduces drag.
- Battery technology: Advanced lithium-ion batteries provide high energy density.
- Energy-saving mode: “Chill” mode limits acceleration and top speed, enhancing efficiency.
Conclusion
Tractive power and energy consumption are crucial factors influencing vehicle performance and sustainability. By understanding the relationship between these elements and implementing innovative strategies, we can drive towards a greener, more energy-efficient future for transportation.
Tips and Tricks
- Monitor driving style using onboard data recorders to identify areas for improvement.
- Plan routes to minimize hills and gradients.
- Consider aerodynamic enhancements such as spoilers and side skirts.
- Explore energy-saving technologies such as adaptive cruise control and lane-keeping assist.
Common Mistakes to Avoid
- Ignoring rolling resistance by neglecting tire maintenance.
- Overestimating engine power and underutilizing the vehicle’s potential efficiency.
- Aggressive driving habits that consume excessive energy.
- Misusing energy-saving modes without understanding their function.